In the early morning of April 30, 35 years ago, I was awakened by a call from Anatoly Dobrynin, a long-time Soviet Ambassador to the United States.
He said General Secretary Mikhail Gorbachev wanted me to come to the Soviet Union to help treat victims of the Chernobyl nuclear power facility accident. I had cabled Gorbachev a few days earlier, offering my assistance.
Last year, I wrote a series of reviews of the HBO Chernobyl miniseries in The Cancer Letter, discussing acute health effects of exposure to acute high dose ionizing radiations, and correcting some inaccuracies in the TV series.
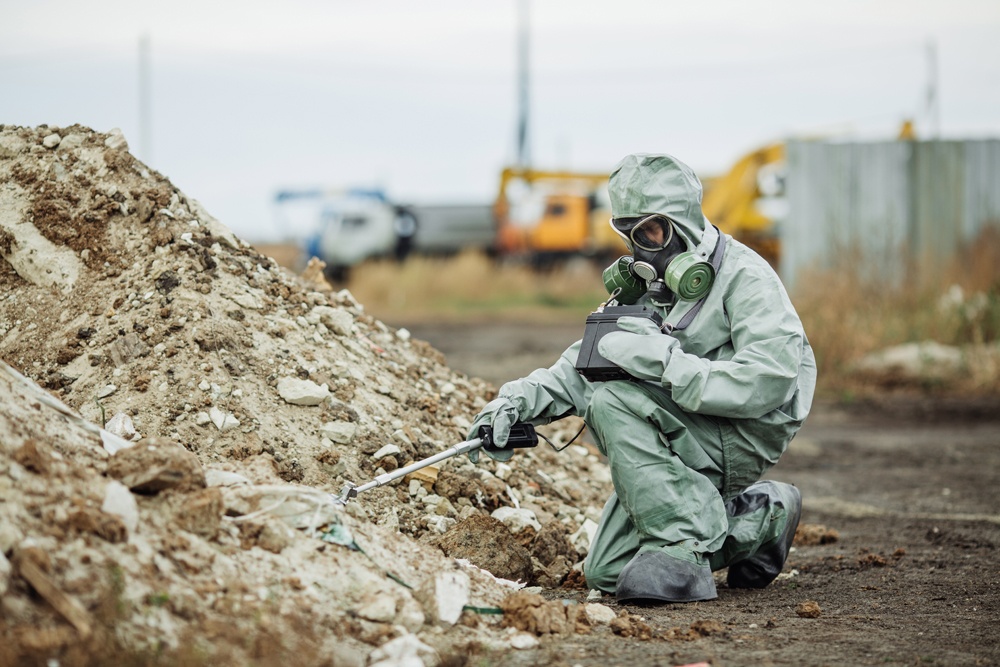
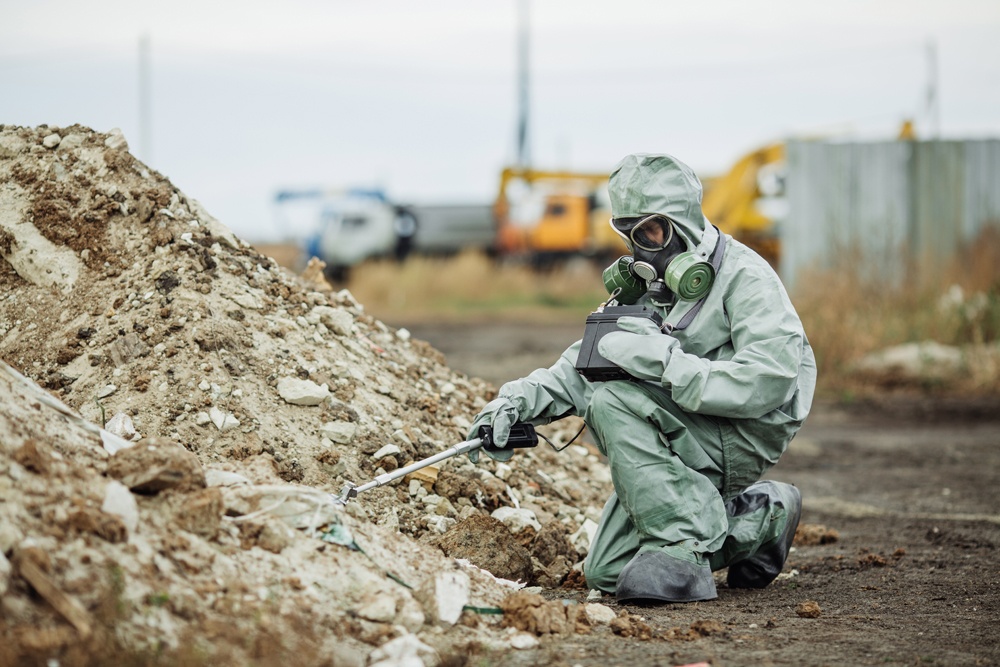
Now, with 35 year follow-up, I update what we know about the long-term health effects, especially cancer risk.
First, some background. What do we know for certain and what is a guess?
The relationship between exposure to ionizing radiations and cancer risk is complex and controversial. Much of what we know is based on data from the A-bomb survivors. In this instance we have reasonably accurate estimates of radiation dose in 94,000 exposed persons and, most importantly, a control cohort of 27,000 residents of Hiroshima and Nagasaki luckily not in the cities when the A-bomb exploded and who were matched for important co-variates such as sex and age with the exposed survivors.
These 121,000 persons have been carefully followed since 1950 with the oldest now 76 years. Having a control cohort allows us to reasonably accurately estimate the proportion of cancers caused by or contributed to by A-bomb radiation exposures.
This risk is obviously dose-related, but, simply put, about one-third of leukemias (absolute number 94) were caused by the A-bomb, but only 10% of solid cancers (absolute number 848).
At doses of 5-100 milliSieverts (a measure of radiation dose) the increased cancer risk to an exposed survivor is about 2%, whereas at doses > 2000 milliSieverts the risk is > 60%. Below, I compare these doses to those received because of the Chernobyl nuclear power facility accident.
The A-bomb data also allow us to determine the briefest interval from radiation exposure to cancer diagnosis. For leukemias, this is about two years, for solid cancers, about 10 years, although most of the increase in solid cancer occurred after 30 years.
These increased risks, especially for solid cancers, remain over a person’s lifetime. Conclusions from the A-bomb survivor studies are mostly confirmed by studies of other radiation-exposed populations such as persons with cancer receiving radiation therapy, radiologists and others.
As important as these data are, there are limitations trying to apply risk estimators from the A-bomb survivors to other radiation exposure scenarios, such as the global population exposed to ionizing radiations from the Chernobyl nuclear power facility accident.
First, the A-bomb survivors were exposed to acute, high-dose whole-body radiations. This contrasts the scenario to billions of people exposed to radiations from the Chernobyl accident, which was (and remains) chronic, low-dose exposure.
Other differences include external exposure versus external and internal exposure, genetic background, and others. As such, one must be cautious in applying estimates based on the A-bomb survivors to exposed persons from the Chernobyl nuclear power facility accident.
Most but not all scientists think there is a linear, no-threshold relationship between radiation dose and cancer risk. The implication is that any excess radiation exposure increases cancer risk. This is not to say one photon can cause cancer, but that one photon increases cancer risk.
The linear, no-threshold relationship is the most conservative interpretation of the data we have and the most protective of public health. However, there are several problems with accepting this hypothesis.
For example, people around the world are exposed to very different background radiation doses. If you live in Denver, your annual background radiation dose is substantially higher than if you live in New York City. Why? Radiation in the soil and rocks, distance from the sun and other factors. However, we do not detect substantial differences in cancer incidences between Denver and New York. Other places in the word such as Ramsar, Iran (radon hot springs), and Guarapari, Brazil (radioactive sand), have 10- or 50-fold greater background radiation doses—with no detectable increase in cancer risk.
The message is the need for caution in applying our radiation risk cancer estimators to relatively low-dose chronic radiation exposure.
Now, back to Chernobyl.
Radiation from the accident spread throughout the Northern Hemisphere, which is home to 6.4 billion people. Cesium-137 released by the Chernobyl accident has a half-life of 30 years meaning it will be a concern for about 300 years (10 half-lives). How does this translate to increased cancer risk?
This requires some background:
The average American is exposed to about 6 milliSieverts (mSv) a year of radiation. About half is naturally-occurring background radiations (the earth’s crust, cosmic radiations, your marble countertops, porcelain teeth etc.). The other one-half is man-made, mostly from radiological studies ordered by physicians, such as computed tomography and positron emission tomography scans.
If we turn to the people living in Ukraine, Belarus and Russia including the 300,000 evacuees and people still living in contaminated lands, the average lifetime excess dose from the Chernobyl accident is about 10-30 milliSieverts.
This dose is equivalent to the background dose received from living three to 10 years in New York City or to five years living in Denver. Put otherwise, this dose is equivalent to someone moving from New York to Denver for three to 10 years.
As you can guess from the above discussion, using this reasoning we would not expect excess cancers from radiation exposures from the Chernobyl accident.
The other way to approach this question is to apply the cancer risk estimator from the A-bomb survivors to the 6.4 billion residents of the Northern Hemisphere. When you do this, even the smallest estimated excess cancer risk translates to millions of excess cancers.
But is applying a risk estimate derived from a very small radiation dose appropriate?
How to resolve this issue?
Let’s look at the data over these 35 years after the Chernobyl accident, keeping in mind that these epidemiological studies are unavoidably flawed: uncontrolled co-variates (increased drinking and smoking in the ex-Soviet Union), ascertainment, biases etc. They are also inappropriately colored by politics: anti- and pro-Russian positions, anti- and pro-nuclear energy positions etc.
First, there is no question there was an extraordinary increase in thyroid cancers in children and adolescents living in Ukraine, Belarus, and Russia, proximal to the accident.
There are about 7,000 excess thyroid cancers, an estimated 100-fold increased incidence, fortunately most not fatal. These were caused by exposure to iodine-131, predominantly from milk consumption. This increased risk continues today, but is decreasing.
A study from NCI scientists published this week in Science reported chromosome translocations resulting in gene fusions were especially common in these children, and typically involved the mitogen-activated protein kinase (MAPK) pathway.
What of other cancers? Leukemias (except CLL) were the first cancers detected after the A-bomb explosions, some occurring within two years, but peaking at about 10 years, and decreasing gradually.
You can think of leukemias after radiation exposure as a canary in a mine used to detect carbon monoxide. If the canary keels over, get out!
Consequently, we were looking carefully for an increase in leukemias in the first 10 years after the Chernobyl accident, but found no convincing evidence. An exception is a report of a modest increase in CLL in persons assigned to mitigate the accident (liquidators, in Russian), who received much higher radiation doses than the general population. No increased leukemias by 10 years was encouraging.
What of solid cancers? We should recall only 10% of cancers in the A-bomb survivors receiving a much higher radiation dose were caused by radiation, an increase which was not detected until about 30 years after exposure and persists.
This means that if there was a Chernobyl-related increase in solid cancers, we should be seeing it now. However, there is only one report of a small increase in solid cancer, breast cancer, again in more heavily-exposed women. Again, good news.
The bottom line is there are few data suggesting that radiation released from Chernobyl increased cancer globally. We must admit, failure to detect an increase does not prove no increase occurred. However, any increase must be below our level of detection.
To put this in context, about 40% of us will develop cancer in our lifetime. Even if there were an increase from Chernobyl related radiation, it would be less than 0.1% using current radiation risk models.
There is also good news regarding birth defects and genetic abnormalities. There are no convincing data of an increase in either. Another study from the NCI, published this week in Science, using next generation sequencing (NGS) to study mother-father pairs exposed to a range of radiation doses from the Chernobyl accident reported no increase in de novo mutations in their children.
Lastly, a word on global climate change, especially in the newly announced U.S. commitment to reduce U.S. carbon emissions by one-half by 2030. Given the increasing demand of electricity in resource-poor and developing countries, there is no reasonable short-term replacement for fossil fuels other than nuclear energy.
There are about 440 nuclear power reactors in operation supplying about 10% of global energy, with 50 under construction worldwide, mostly in Asia, but including several in oil rich countries such as the UAE. Certainly, we should focus on conservation, solar, hydro-electric, geo-thermal and other renewable energy sources.
However, none can fully meet our energy demand. Nuclear energy is a reasonable, perhaps necessary short-term alternative energy source. But safeguards are needed. And we cannot escape the link between peaceful and non-peaceful uses of nuclear technology.
In summary, the Chernobyl nuclear power facility accident was a global tragedy. I focused on the health consequences, which were small, but we must not forget the social, psychological, and economic consequences of the accident.
The Chernobyl accident was preventable—as was the Fukushima accident. We now have smarter, safer nuclear reactors and lessons learned from these accidents.
Now is the time for the U.S. and the world to re-evaluate the potential role of nuclear energy in helping us deal with climate change.
Gale is a co-author (with Eric Lax) of “Radiation: What It Is, What You Need to Know,” and (with Thomas Hauser ) of “Chernobyl: The Final Warning.”
From NCI: Research teams use genomic tools to explore long-term effects of Chernobyl radiation
In two landmark studies, researchers have used cutting-edge genomic tools to investigate the potential health effects of exposure to ionizing radiation from the 1986 accident at the Chernobyl nuclear power plant.
One study found no evidence that radiation exposure to parents resulted in new genetic changes being passed from parent to child. The second study documented the genetic changes in the tumors of people who developed thyroid cancer after being exposed as children or fetuses to the radiation released by the accident.
The findings, published around the 35th anniversary of the disaster, are from international teams of investigators led by researchers at NCI. The studies were published online in Science on April 22.
“Scientific questions about the effects of radiation on human health have been investigated since the atomic bombings of Hiroshima and Nagasaki and have been raised again by Chernobyl and by the nuclear accident that followed the tsunami in Fukushima, Japan,” Stephen J. Chanock, director of NCI’s Division of Cancer Epidemiology and Genetics, said in a statement. “In recent years, advances in DNA sequencing technology have enabled us to begin to address some of the important questions, in part through comprehensive genomic analyses carried out in well-designed epidemiological studies.”
The Chernobyl accident exposed millions of people in the surrounding region to radioactive contaminants. Studies have provided much of today’s knowledge about cancers caused by radiation exposures from nuclear power plant accidents. The new research builds on this foundation using next-generation DNA sequencing and other genomic characterization tools to analyze biospecimens from people in Ukraine who were affected by the disaster.
The first study investigated the long-standing question of whether radiation exposure results in genetic changes that can be passed from parent to offspring, as has been suggested by some studies in animals. To answer this question, Chanock and his colleagues analyzed the complete genomes of 130 people born between 1987 and 2002 and their 105 mother-father pairs.
One or both of the parents had been workers who helped clean up from the accident or had been evacuated because they lived in close proximity to the accident site. Each parent was evaluated for protracted exposure to ionizing radiation, which may have occurred through the consumption of contaminated milk (that is, milk from cows that grazed on pastures that had been contaminated by radioactive fallout). The mothers and fathers experienced a range of radiation doses.
The researchers analyzed the genomes of adult children for an increase in a particular type of inherited genetic change known as de novo mutations. De novo mutations are genetic changes that arise randomly in a person’s gametes (sperm and eggs) and can be transmitted to their offspring but are not observed in the parents.
For the range of radiation exposures experienced by the parents in the study, there was no evidence from the whole-genome sequencing data of an increase in the number or types of de novo mutations in their children born between 46 weeks and 15 years after the accident.
The number of de novo mutations observed in these children were highly similar to those of the general population with comparable characteristics. As a result, the findings suggest that the ionizing radiation exposure from the accident had a minimal, if any, impact on the health of the subsequent generation.
“We view these results as very reassuring for people who were living in Fukushima at the time of the accident in 2011,” Chanock said. “The radiation doses in Japan are known to have been lower than those recorded at Chernobyl.”
In the second study, researchers used next-generation sequencing to profile the genetic changes in thyroid cancers that developed in 359 people exposed as children or in utero to ionizing radiation from radioactive iodine (I-131) released by the Chernobyl nuclear accident and in 81 unexposed individuals born more than nine months after the accident. Increased risk of thyroid cancer has been one of the most important adverse health effects observed after the accident.
The energy from ionizing radiation breaks the chemical bonds in DNA, resulting in a number of different types of damage. The new study highlights the importance of a particular kind of DNA damage that involves breaks in both DNA strands in the thyroid tumors. The association between DNA double-strand breaks and radiation exposure was stronger for children exposed at younger ages.
Next, the researchers identified the candidate “drivers” of the cancer in each tumor — the key genes in which alterations enabled the cancers to grow and survive. They identified the drivers in more than 95% of the tumors. Nearly all the alterations involved genes in the same signaling pathway, called the mitogen-activated protein kinase (MAPK) pathway, including the genes BRAF, RAS, and RET.
The set of affected genes is similar to what has been reported in previous studies of thyroid cancer. However, the researchers observed a shift in the distribution of the types of mutations in the genes. Specifically, in the Chernobyl study, thyroid cancers that occurred in people exposed to higher radiation doses as children were more likely to result from gene fusions (when both strands of DNA are broken and then the wrong pieces are joined back together), whereas those in unexposed people or those exposed to low levels of radiation were more likely to result from point mutations (single base-pair changes in a key part of a gene).
The results suggest that DNA double-strand breaks may be an early genetic change following exposure to radiation in the environment that subsequently enables the growth of thyroid cancers. Their findings provide a foundation for further studies of radiation-induced cancers, particularly those that involve differences in risk as a function of both dose and age, the researchers added.
“An exciting aspect of this research was the opportunity to link the genomic characteristics of the tumor with information about the radiation dose — the risk factor that potentially caused the cancer,” said Lindsay M. Morton, deputy chief of the Radiation Epidemiology Branch in DCEG, who led the study.
“The Cancer Genome Atlas set the standard for how to comprehensively profile tumor characteristics,” Morton said. “We extended that approach to complete the first large genomic landscape study in which the potential carcinogenic exposure was well-characterized, enabling us to investigate the relationship between specific tumor characteristics and radiation dose.”
She noted that the study was made possible by the creation of the Chernobyl Tissue Bank about two decades ago — long before the technology had been developed to conduct the kind of genomic and molecular studies that are common today.
“These studies represent the first time our group has done molecular studies using the biospecimens that were collected by our colleagues in Ukraine,” Morton said. “The tissue bank was set up by visionary scientists to collect tumor samples from residents in highly contaminated regions who developed thyroid cancer. These scientists recognized that there would be substantial advances in technology in the future, and the research community is now benefiting from their foresight.”